An Analytical Perspective on the Competition Between Air Defense Systems and Guided Air-to-Ground Munitions
Dr. Feridun TAŞDAN - Western Illinois University
Introduction: Until today, conventional wars begin primarily in the air, and after the establishment of air superiority, the remaining stages of the war continue as the destruction of critical land and sea targets of the other side. According to the forecasts for the next 10-20 years, it will be extremely challenging to gain air superiority and to approach hostile targets protected by modern and integrated air defense systems using classical methods. New and improved systems are being developed that can intercept not only warplanes but also long-range cruise missiles and even short-range munitions such as JDAM before reaching their targets. For example, it has become possible to decrease the accuracy of JDAM-like bombs by jamming their GPS signals or destroy them mid-air with anti-aircraft guns using programmable air-burst smart rounds. To protect themselves and penetrate the enemy’s highly protected airspace, warplanes must have certain capabilities such as stealth, electronic support, jamming, data fusion, and effective command control. Likewise, long-range cruise missiles or short-range (around 15km) laser-guided smart munitions classified as Precision Guided Munitions (OGM), will have to operate in much more challenging conditions now. Because thanks to the advancements in sensor and missile technologies, different air defense systems (low, medium, and high altitude) under the management of modern and integrated command control systems with a high hit probability, can now engage various types of air threats more effectively.
During the 1990 Gulf War and then the 2003 invasion of Iraq, the U.S. Air Force (USAF) used approximately 1.5 Precision Guided (Smart) Munitions per target, considering that the probability of PGMs reaching their target is over 95%. On the other hand, if we look at the sortie to target ratio in World War II, approximately 1,000 bombers and 9,000 unguided (dumb) bombs were used to kill a ground target. During the Vietnam War in the 1970s, about 30 sorties and 176 unguided bombs had to be used for each target. By the 1990s, the GBU-10/12 series Laser Guided Bombs (LGB) started to be widely used, thus allowing one munition/sortie to be used against one ground target. Today, in parallel with the advancements in GPS and other guidance technologies, 80 different bombs can be used against 80 different ground targets in a single sortie by the B-2 or other bomber aircraft of the U.S. Air Force. But what about the situation in 2020 and beyond? How long will short or long-range smart bombs, which use several different guidance techniques and are much more developed than in the 2000s, be able to maintain their dominant effects on targets? The answer is that combat technologies are not developed on a single axis, and similar advancements can now be observed in air defense systems and other passive interception systems.
Estimating the probability of Precision Guided Munitions (PGMs) reaching their targets without being hit in current and future air-to-ground operations is quite challenging. For example, numerous factors such as the capabilities of the hostile air defense systems (reaction time, radar, the effectiveness of E/O systems, ECM resistance, etc.), training & readiness levels of the soldiers who use these systems, and maintenance infrastructure can affect the success of the hit and the destruction of the target.
Additionally, it would not be wrong to predict that today’s developed and currently developed air defense systems will have AESA radars using advanced Gallium Nitride (GaN) semiconductor technology, R.F. signal processors with high computing capability, passive IIR guidance capability, ECM resistance, and a well-integrated layered radar network. In addition to air-breathing targets, the efficiency of air defense systems will continue to increase against guided munitions with low radar cross-section (RCS). For example, Israel’s combat-proven Iron Dome system, which was actively tested in actual battlefield conditions, is stated to have a successful engagement rate of over 90%. The hit-to-kill system is designed to intercept and destroy short-range Katyusha type, or longer-range 122mm rockets and artillery shells fired by Hezbollah. It is possible to purchase and modify a similar system by another country and use it to counter cruise missiles and other sensitive guided munitions in case of an attack on an airbase. Manufacturers of air defense systems such as Skyshield, C-RAM, and Pantsir S1 insist that their products are effective against all air-breathing targets, including precision-guided munitions. Military officials and various strategic research centers in Washington DC now state that military targets protected by these systems cannot be destroyed with only one sortie or one munition.
An Analytical Look at The Competition Between Air Defense Systems (ADS) and Precision Guided Munitions (PGM)
In recent years, during the airstrikes conducted in the Syrian territory, Israel employed different munition types such as Delilah, Spice, or JDAM against various ground targets that Israel perceived as a threat. Similarly, in April 2017, the United States launched 59 Tomahawk cruise missiles from the Mediterranean against 20 different targets at Shayrat Airbase controlled by the Syrian government. Also, in 2018, NATO countries led by the United States carried out a missile strike on another location in Syria with a total of 109 Scalp, Tomahawk, and JASSM-type cruise missiles. The common point of these attacks is the use of many cruise missiles despite the small number of targets. In September 2019, the attack carried out with a total of 20-25 drones and missiles against two different Saudi Aramco oil refineries in the west of Riyadh caused significant damage to the facilities despite the existence of a Saudi Patriot battery tasked with protecting the facilities. The attack is believed to have been carried out using a piston-powered drone named Afif, which is presumed to be the Iranian copy of Israeli-made Harpy, and a mini turbojet-powered cruise missile named Quds-1 supposedly produced in Yemen with the help of Iran.
Based on these examples, let us try to answer how many PGMs should be used to destroy a target/target group with analytical methods. At the first stage, the mathematical comparison of the relationship between PGM and SAM will be evaluated regardless of the time variable. Additionally, it was assumed that PGMs were launched from aircraft or ships to their targets without engaging in air-to-air combat.
Hitting/intercepting an aerial target with a Surface-to-Air Missile (SAM) system can be considered a two-result probability experiment. Based on whether the air target is destroyed or not, we can interpret the result of the experiment as the Air Defense System was successful or failed. For example, let us assume the hit/interception probability of air targets entering the airspace protected by an air defense system (it can be a single mobile system or a battery consisting of several systems) is 80% (i.e., the successful interception probability of the SAM battery is ph=0.80). In case of failure, the Air Defense System will miss its target with qh=1-ph=0.20 probability, and it will be possible for the Precision Guided Munition to reach its target with a 20% chance. In short, the Air Defense System’s failure to protect the target position indicates that a certain number of smart bombs will be able to reach its target.
Let us assume that n PGMs have been launched from a fighter jet or other ground or naval systems independently of each other to reach a targeted region/point. Suppose that variable X has a binomial probability distribution as a coincidence variable and indicates the number of PGMs that has successfully reached its target. Here we can consider the parameters of the binomial probability distribution as n (total number of PGMs launched independently), ph (probability of successful SAM system interception, i.e., the probability of PGM failure), and qh=1-ph (probability of each PGM reaching their target, probability of SAM system failure) respectively. In this case, we can accept P(X ≥ 1) = 1 - P(X = 0) using the binomial probability distribution function. In the second stage, we can expect the P(X ≥ 1) value (probability of at least one PGM to reach its target successfully) to be a minimum probability value. Assuming that this minimum probability value will be the pk, the relationship between the n and the probabilities of pk and ph is n ≤ log(1-pk)/log(ph). We can explain the meaning of the n value above as at least how many PGMs should be used, if we consider the probability of at least one PGM reaching its target is pk, and the probability of SAM systems successfully intercepting PGMs is ph.
As illustrated in Figure-1, if we consider the probability of at least one PGM reaching its target as pk=0.97, and the likelihood of SAM systems successfully intercepting PGMs ph=0.50, at least n=5 PGMs will be required to hit the target. Again, if the Air Defense System’s interception success is accepted as ph=0.80, n=16 PGMs should be launched to a single target so that at least one PGM can reach its target with a probability of 97% and above. This is explained in Figure-1 shown above. As can be seen, as the successful hit probability (ph) of the SAM system increases, the number of PGMs required to destroy the target increases exponentially. It will be quite a challenge to penetrate a defense line consisting of modern Air Defense Systems that operate integrally, as can be seen in today’s conventional wars.
Considering the possible pk and ph values together, the total PGM launch matrix required for at least one PGM to reach its target is estimated in
In the matrix above, the probability of at least one PGM reaching its target is defined as pk, and the probability of successful interception of the air defense system is defined as ph, and the number of PGMs that must be launched to the target for at least one hit is estimated . For example, when an Air Defense System’s probability of successful interception is considered ph=0.75 under the condition of at least pk=0.95 probability, at least n=10 PGMs will be required for at least one PGM to reach its target. If we look at the maximum limits (99% Air Defense System interception rate and 99% PGM hit rate), we can see extremely high results that approximately n=458 guided munitions must be used. The 99% success rate for both sides means that the events are certain to happen, so an unusually high number of PGMs is required for at least one hit.
The number of PGMs that should be used to hit and destroy the target will also determine the number of sorties. As can be seen from Table-1, an Air Defense System with high ph will also require a high number of PGMs. For example, if we accept our criteria as ph=0.75 and pk=0.95, at least 10 PGMs will need to be launched so that at least one of them can reach its target. In case of using the lightest 500lb GBU-12 LGB or GBU-38 JDAM, each aircraft will need to load four bombs, and 2.5-3 sorties will be required. If we want to use a long-range cruise missile such as SOM, it means that about 5 F-16 aircraft sorties (each plane can carry 2 SOMs) need to be conducted against a single target.
This article aims to show readers the analytical relationship between Precision Guided Munitions and Air Defense Systems in a simplified form. Of course, it is almost impossible for an Air Defense System to have a 99% success rate in a real conflict. Moreover, the number of missiles ready to fire in a SAM battery will never reach numbers like 100-200. In real-life conditions, success rates of Air Defense Systems can be reduced by using different tactics such as electronic warfare, decoys, and surprise attacks.
Reviewing the Relationship Between Precision Guided Munitions and Air Defense Systems with the Queuing Theory: We can compare the launch of a Precision Guided Munition (PGM) and the process of reaching its target to a customer who walks toward the checkout at a market. On the other hand, the Air Defense System can be considered as the cashier serving its customers. Since the relationship between the PGM flying towards its target and the SAM system, which tries to engage and intercept it, is time-dependent, it will be regarded as a scholastic process (Markov Process) and will be examined using Queueing Theory.
Suppose there are s Air Defense Systems (s service stations) that protect the target area. We can assume that the average engagement rate (service rate) of each Air Defense System has the µ parameter for each system. We can accept the average arrival rate of PGMs as λ. If we accept the queue discipline as first come, first served, the SAM system will prioritize the first detected PGM and start the engagement process accordingly. Let us suppose that the number of arrivals of PGMs matches the Poisson distribution with the λ parameter, and the SAM system engagement rate (service rate) matches the Exponential distribution with the µ parameter. Considering the arrival of PGM as birth, and the interception of the target by the SAM system as death, our queueing model, in simplified form, can be defined as Birth and Death model with s service stations described by M/M/s code. Here we will give the mathematical details of the formulas as simplified as possible.
One of the factors that determine the system’s performance in Queuing Theory is the relationship between the arrival rate and the service rate. This ratio is the ρ= λ/(s*µ) equation called traffic density. If the traffic density (ρ) ratio is ρ > 1, it means that more customers come to the service station than the system can handle. In the relationship between PGM and SAM systems, ρ > 1 means the saturation of the SAM system with s firing units. Then the question to be asked is how many PGMs should be used against the target at the same time (Time on Target) to saturate the Air Defense System. As can be understood from the ρ= λ/(s*µ) formula, the system will reach saturation according to the λ, µ, and s values.
For example, let us assume that there are four air defense systems in the target area that can operate independently with 360-degree engagement capability, and the average engagement rate of each system is µ=5. In this case, to achieve ρ > 1, the service rate will be λ/(s*µ)>1, and the result will be λ>(s*µ)=20. This means that to saturate the Air Defense System, λ > 20 number of PGMs should be on the target (ToT) at the same time (within 1 minute). In short, during the planning phase of an operation, the types and number of munitions to be used, as well as the need for electronic warfare, are determined based on the information about the Air Defense System located in the operation area.
In a possible scenario (Table-2), let us analyze a PGM attack on a target position using Queue Theory, assuming that the area is protected by 4 Pantsir-S2 (or TOR-M2) firing units. Let us suppose that according to the fundamental rule of Queueing Theory (first come, first serve), the first PGM will be engaged without waiting. Although this point air defense system, which operates according to the CLOS (command line of sight) guidance principle, has 360-degree engagement capability and a 40km range S-band search radar, with a 30km range EHF band engagement radar, and an IR/TV guidance system has CLOS guidance capability in a 90-degree sector only. Therefore, let us assume that at least 4 Pantsir-S2 firing units protect the target area against PGM attacks (to have 360-degree engagement capability) that can come from different directions at the same time. The unit of time will be considered in minutes. As parameters, let us assume that PGM time on target ratio is λ=(5,10,15,20,25,30), the average target engagement rate of each Pantsir unit is µ=8, the number of Pantsir units is s=4, engagement capacity (density) is ρ=λ/(s*µ), the number of PGMs waiting in the queue before the engagement is Lq=E[Qq], and queue time for engagement (seconds) is Wq=E[Tq]. The following table was created using the M/M/s birth-death model and the R software program by giving the estimated values to these parameters.
As it can be understood from Table-2, during a PGM attack on a target protected by 4 Pantsir-S2 units, if the number of PGMs required to reach the target is between 25 <λ<30, the engagement capacity (density) will be between 0.781<ρ<0.938. If λ=25, then Lq=2.011, meaning the number of PGMs that have not been engaged yet (the number of PGMs waiting in the queue for engagement). If Wq=4.8 sec, it shows the expected time for engagement in the queue. Especially in the case of λ=30 PGMs, it is seen that at least Lq=12.95~13 PGMs will wait in the queue for engagement, and the average waiting time of these PGMs will be approximately Wq=25.95~26 seconds. Naturally, PGMs that have not yet been engaged (waiting in the queue) will not remain in the air, like a market customer, and the target will be destroyed in a few seconds. If λ>32 (in case of using 32 PGMs or more), the system will be completely saturated (ρ>1). Naturally, real war conditions are very different, and the theoretical figures described here may not reflect reality. For example, it may be possible to use decoys instead of real PGMs, or the rapid engagement capability of the Pantsir units can be abbreviated with remote electronic jamming. For example, if Pantsir’s engagement rate per minute is reduced to 2-3 (such as µ=2), the system can be saturated using a much smaller number of PGMs or decoys. Using low RCS munitions such as Delilah, decoys, electronic countermeasures (ECM), and combat tactics such as surprise attacks may play a critical role in Israel’s success against Pantsir systems in Syria in recent years.
Conclusion and Suggestions
In peacetime, the existence and models of air defense and other passive interception systems owned by hostile countries should be identified, and countermeasures should be developed against these systems. In short, it is necessary to have a robust intelligence network and advanced E.W. systems to decrease the effectiveness (reducing the probability of interception) of enemy Air Defense Systems. These E.W. systems include long-range electronic support and countermeasure systems for signal intelligence (SIGINT), land/ship-based electronic support/countermeasure systems, or signal blocking devices (jammers). Returning to the matrix above in Table-3, when we reduce the probability of successful interception of the air defense system from 75% to 50%, the number of PGMs required for at least one hit with a 95% success rate decreases from 10 to 4. Likewise, in addition to the E.W. systems, air-launched decoy missiles such as the ADM-160 MALD (Miniature Air-Launched Decoy/USA) and ITALD (Improved Tactical Air-Launched Decoy/Israel) can also be deployed to trick hostile Air Defense Systems into spending their missiles and ammunition on false targets instead of real targets. Thus, real munitions can be used in the second wave that will follow immediately.
Various factors such as stealth-configured specialized structure geometry and the use of RAM paint and composite materials should also be considered to reduce the radar cross-sections of cruise missiles like SOM as much as possible. Secondly, cruise missiles with advanced guidance systems such as SOM may be upgraded with new features such as swarming capability, networking, and smart flight management systems that can monitor each missile’s status and automatically direct them to another nearby target that is not hit yet.
While countries such as the USA and Israel are developing different sized guided munition options, they have started to prioritize small diameter guided munitions. The USAF and US NAVY are currently investing in 120kg SDB (Small Diameter Bomb) munitions that use two or three different types of guidance, such as GBU-39 (SDB-I) and GBU-53 (SDB-II). Thanks to their control surfaces and guidance systems, these bombs can reach a range of 100 km when launched at medium-high altitudes. An F-16 aircraft can carry a total of 8 SDB-I/II ammunition with two BRU-61 ejector racks, each of which can be loaded with four bombs. Similarly, Israel has developed Spice 250 munition with multiple guidance (INS/GPS and E/O guidance) from the Spice family. It weighs 250lb (122kg) and a total of eight bombs can be carried by an F-16 aircraft.
In parallel with these developments, Turkey is also developing a small-diameter/light-weight bomb with similar guidance characteristics as the US GBU-39 SDB-I munition. Co-developed by Aselsan/SAGE, this munition, which weighs around 125kg, will have a range of 100km when launched from high altitudes thanks to its diamond-shaped folded wings like the GBU-39 SDB-I bomb. Another significant advantage of using SDBs is that it allows carrying a higher amount of munition, enabling multiple target engagements in a single sortie.
Furthermore, to provide solutions at the operational level, it is necessary to carefully monitor the swarm UAVs and smart munitions that are still in the R&D process. With the introduction of this technology, air defense will no longer be possible with conventional missile/gun systems. It will be inevitable for Air Defense Systems to adapt directed-energy weapons that utilize lasers or electromagnetic (railgun) technologies to counter swarm drone attacks.
On the other hand, in peacetime, PGM stocks should be prepared in different varieties (short, medium, or long-range) and numbers according to the priority and type (such as hard targets, soft targets, or fortifications) of targets to be destroyed. For example, while stocking a large amount of LGB/JDAM type short-range (under 20km) munitions, it is also necessary to have a certain number of medium-range (20-100km) PGMs, and finally, a sufficient number of long-range (over 100km) cruise missiles (air, ground, or sea-launched) are required to hit very high-value critical targets protected by multi-layered Air Defense Systems
www.defenceturkey.com
Dr. Feridun TAŞDAN - Western Illinois University
Introduction: Until today, conventional wars begin primarily in the air, and after the establishment of air superiority, the remaining stages of the war continue as the destruction of critical land and sea targets of the other side. According to the forecasts for the next 10-20 years, it will be extremely challenging to gain air superiority and to approach hostile targets protected by modern and integrated air defense systems using classical methods. New and improved systems are being developed that can intercept not only warplanes but also long-range cruise missiles and even short-range munitions such as JDAM before reaching their targets. For example, it has become possible to decrease the accuracy of JDAM-like bombs by jamming their GPS signals or destroy them mid-air with anti-aircraft guns using programmable air-burst smart rounds. To protect themselves and penetrate the enemy’s highly protected airspace, warplanes must have certain capabilities such as stealth, electronic support, jamming, data fusion, and effective command control. Likewise, long-range cruise missiles or short-range (around 15km) laser-guided smart munitions classified as Precision Guided Munitions (OGM), will have to operate in much more challenging conditions now. Because thanks to the advancements in sensor and missile technologies, different air defense systems (low, medium, and high altitude) under the management of modern and integrated command control systems with a high hit probability, can now engage various types of air threats more effectively.
During the 1990 Gulf War and then the 2003 invasion of Iraq, the U.S. Air Force (USAF) used approximately 1.5 Precision Guided (Smart) Munitions per target, considering that the probability of PGMs reaching their target is over 95%. On the other hand, if we look at the sortie to target ratio in World War II, approximately 1,000 bombers and 9,000 unguided (dumb) bombs were used to kill a ground target. During the Vietnam War in the 1970s, about 30 sorties and 176 unguided bombs had to be used for each target. By the 1990s, the GBU-10/12 series Laser Guided Bombs (LGB) started to be widely used, thus allowing one munition/sortie to be used against one ground target. Today, in parallel with the advancements in GPS and other guidance technologies, 80 different bombs can be used against 80 different ground targets in a single sortie by the B-2 or other bomber aircraft of the U.S. Air Force. But what about the situation in 2020 and beyond? How long will short or long-range smart bombs, which use several different guidance techniques and are much more developed than in the 2000s, be able to maintain their dominant effects on targets? The answer is that combat technologies are not developed on a single axis, and similar advancements can now be observed in air defense systems and other passive interception systems.
Estimating the probability of Precision Guided Munitions (PGMs) reaching their targets without being hit in current and future air-to-ground operations is quite challenging. For example, numerous factors such as the capabilities of the hostile air defense systems (reaction time, radar, the effectiveness of E/O systems, ECM resistance, etc.), training & readiness levels of the soldiers who use these systems, and maintenance infrastructure can affect the success of the hit and the destruction of the target.
Additionally, it would not be wrong to predict that today’s developed and currently developed air defense systems will have AESA radars using advanced Gallium Nitride (GaN) semiconductor technology, R.F. signal processors with high computing capability, passive IIR guidance capability, ECM resistance, and a well-integrated layered radar network. In addition to air-breathing targets, the efficiency of air defense systems will continue to increase against guided munitions with low radar cross-section (RCS). For example, Israel’s combat-proven Iron Dome system, which was actively tested in actual battlefield conditions, is stated to have a successful engagement rate of over 90%. The hit-to-kill system is designed to intercept and destroy short-range Katyusha type, or longer-range 122mm rockets and artillery shells fired by Hezbollah. It is possible to purchase and modify a similar system by another country and use it to counter cruise missiles and other sensitive guided munitions in case of an attack on an airbase. Manufacturers of air defense systems such as Skyshield, C-RAM, and Pantsir S1 insist that their products are effective against all air-breathing targets, including precision-guided munitions. Military officials and various strategic research centers in Washington DC now state that military targets protected by these systems cannot be destroyed with only one sortie or one munition.
An Analytical Look at The Competition Between Air Defense Systems (ADS) and Precision Guided Munitions (PGM)
In recent years, during the airstrikes conducted in the Syrian territory, Israel employed different munition types such as Delilah, Spice, or JDAM against various ground targets that Israel perceived as a threat. Similarly, in April 2017, the United States launched 59 Tomahawk cruise missiles from the Mediterranean against 20 different targets at Shayrat Airbase controlled by the Syrian government. Also, in 2018, NATO countries led by the United States carried out a missile strike on another location in Syria with a total of 109 Scalp, Tomahawk, and JASSM-type cruise missiles. The common point of these attacks is the use of many cruise missiles despite the small number of targets. In September 2019, the attack carried out with a total of 20-25 drones and missiles against two different Saudi Aramco oil refineries in the west of Riyadh caused significant damage to the facilities despite the existence of a Saudi Patriot battery tasked with protecting the facilities. The attack is believed to have been carried out using a piston-powered drone named Afif, which is presumed to be the Iranian copy of Israeli-made Harpy, and a mini turbojet-powered cruise missile named Quds-1 supposedly produced in Yemen with the help of Iran.
Based on these examples, let us try to answer how many PGMs should be used to destroy a target/target group with analytical methods. At the first stage, the mathematical comparison of the relationship between PGM and SAM will be evaluated regardless of the time variable. Additionally, it was assumed that PGMs were launched from aircraft or ships to their targets without engaging in air-to-air combat.
Hitting/intercepting an aerial target with a Surface-to-Air Missile (SAM) system can be considered a two-result probability experiment. Based on whether the air target is destroyed or not, we can interpret the result of the experiment as the Air Defense System was successful or failed. For example, let us assume the hit/interception probability of air targets entering the airspace protected by an air defense system (it can be a single mobile system or a battery consisting of several systems) is 80% (i.e., the successful interception probability of the SAM battery is ph=0.80). In case of failure, the Air Defense System will miss its target with qh=1-ph=0.20 probability, and it will be possible for the Precision Guided Munition to reach its target with a 20% chance. In short, the Air Defense System’s failure to protect the target position indicates that a certain number of smart bombs will be able to reach its target.
Let us assume that n PGMs have been launched from a fighter jet or other ground or naval systems independently of each other to reach a targeted region/point. Suppose that variable X has a binomial probability distribution as a coincidence variable and indicates the number of PGMs that has successfully reached its target. Here we can consider the parameters of the binomial probability distribution as n (total number of PGMs launched independently), ph (probability of successful SAM system interception, i.e., the probability of PGM failure), and qh=1-ph (probability of each PGM reaching their target, probability of SAM system failure) respectively. In this case, we can accept P(X ≥ 1) = 1 - P(X = 0) using the binomial probability distribution function. In the second stage, we can expect the P(X ≥ 1) value (probability of at least one PGM to reach its target successfully) to be a minimum probability value. Assuming that this minimum probability value will be the pk, the relationship between the n and the probabilities of pk and ph is n ≤ log(1-pk)/log(ph). We can explain the meaning of the n value above as at least how many PGMs should be used, if we consider the probability of at least one PGM reaching its target is pk, and the probability of SAM systems successfully intercepting PGMs is ph.
As illustrated in Figure-1, if we consider the probability of at least one PGM reaching its target as pk=0.97, and the likelihood of SAM systems successfully intercepting PGMs ph=0.50, at least n=5 PGMs will be required to hit the target. Again, if the Air Defense System’s interception success is accepted as ph=0.80, n=16 PGMs should be launched to a single target so that at least one PGM can reach its target with a probability of 97% and above. This is explained in Figure-1 shown above. As can be seen, as the successful hit probability (ph) of the SAM system increases, the number of PGMs required to destroy the target increases exponentially. It will be quite a challenge to penetrate a defense line consisting of modern Air Defense Systems that operate integrally, as can be seen in today’s conventional wars.
Considering the possible pk and ph values together, the total PGM launch matrix required for at least one PGM to reach its target is estimated in
In the matrix above, the probability of at least one PGM reaching its target is defined as pk, and the probability of successful interception of the air defense system is defined as ph, and the number of PGMs that must be launched to the target for at least one hit is estimated . For example, when an Air Defense System’s probability of successful interception is considered ph=0.75 under the condition of at least pk=0.95 probability, at least n=10 PGMs will be required for at least one PGM to reach its target. If we look at the maximum limits (99% Air Defense System interception rate and 99% PGM hit rate), we can see extremely high results that approximately n=458 guided munitions must be used. The 99% success rate for both sides means that the events are certain to happen, so an unusually high number of PGMs is required for at least one hit.
The number of PGMs that should be used to hit and destroy the target will also determine the number of sorties. As can be seen from Table-1, an Air Defense System with high ph will also require a high number of PGMs. For example, if we accept our criteria as ph=0.75 and pk=0.95, at least 10 PGMs will need to be launched so that at least one of them can reach its target. In case of using the lightest 500lb GBU-12 LGB or GBU-38 JDAM, each aircraft will need to load four bombs, and 2.5-3 sorties will be required. If we want to use a long-range cruise missile such as SOM, it means that about 5 F-16 aircraft sorties (each plane can carry 2 SOMs) need to be conducted against a single target.
This article aims to show readers the analytical relationship between Precision Guided Munitions and Air Defense Systems in a simplified form. Of course, it is almost impossible for an Air Defense System to have a 99% success rate in a real conflict. Moreover, the number of missiles ready to fire in a SAM battery will never reach numbers like 100-200. In real-life conditions, success rates of Air Defense Systems can be reduced by using different tactics such as electronic warfare, decoys, and surprise attacks.
Reviewing the Relationship Between Precision Guided Munitions and Air Defense Systems with the Queuing Theory: We can compare the launch of a Precision Guided Munition (PGM) and the process of reaching its target to a customer who walks toward the checkout at a market. On the other hand, the Air Defense System can be considered as the cashier serving its customers. Since the relationship between the PGM flying towards its target and the SAM system, which tries to engage and intercept it, is time-dependent, it will be regarded as a scholastic process (Markov Process) and will be examined using Queueing Theory.
Suppose there are s Air Defense Systems (s service stations) that protect the target area. We can assume that the average engagement rate (service rate) of each Air Defense System has the µ parameter for each system. We can accept the average arrival rate of PGMs as λ. If we accept the queue discipline as first come, first served, the SAM system will prioritize the first detected PGM and start the engagement process accordingly. Let us suppose that the number of arrivals of PGMs matches the Poisson distribution with the λ parameter, and the SAM system engagement rate (service rate) matches the Exponential distribution with the µ parameter. Considering the arrival of PGM as birth, and the interception of the target by the SAM system as death, our queueing model, in simplified form, can be defined as Birth and Death model with s service stations described by M/M/s code. Here we will give the mathematical details of the formulas as simplified as possible.
One of the factors that determine the system’s performance in Queuing Theory is the relationship between the arrival rate and the service rate. This ratio is the ρ= λ/(s*µ) equation called traffic density. If the traffic density (ρ) ratio is ρ > 1, it means that more customers come to the service station than the system can handle. In the relationship between PGM and SAM systems, ρ > 1 means the saturation of the SAM system with s firing units. Then the question to be asked is how many PGMs should be used against the target at the same time (Time on Target) to saturate the Air Defense System. As can be understood from the ρ= λ/(s*µ) formula, the system will reach saturation according to the λ, µ, and s values.
For example, let us assume that there are four air defense systems in the target area that can operate independently with 360-degree engagement capability, and the average engagement rate of each system is µ=5. In this case, to achieve ρ > 1, the service rate will be λ/(s*µ)>1, and the result will be λ>(s*µ)=20. This means that to saturate the Air Defense System, λ > 20 number of PGMs should be on the target (ToT) at the same time (within 1 minute). In short, during the planning phase of an operation, the types and number of munitions to be used, as well as the need for electronic warfare, are determined based on the information about the Air Defense System located in the operation area.
In a possible scenario (Table-2), let us analyze a PGM attack on a target position using Queue Theory, assuming that the area is protected by 4 Pantsir-S2 (or TOR-M2) firing units. Let us suppose that according to the fundamental rule of Queueing Theory (first come, first serve), the first PGM will be engaged without waiting. Although this point air defense system, which operates according to the CLOS (command line of sight) guidance principle, has 360-degree engagement capability and a 40km range S-band search radar, with a 30km range EHF band engagement radar, and an IR/TV guidance system has CLOS guidance capability in a 90-degree sector only. Therefore, let us assume that at least 4 Pantsir-S2 firing units protect the target area against PGM attacks (to have 360-degree engagement capability) that can come from different directions at the same time. The unit of time will be considered in minutes. As parameters, let us assume that PGM time on target ratio is λ=(5,10,15,20,25,30), the average target engagement rate of each Pantsir unit is µ=8, the number of Pantsir units is s=4, engagement capacity (density) is ρ=λ/(s*µ), the number of PGMs waiting in the queue before the engagement is Lq=E[Qq], and queue time for engagement (seconds) is Wq=E[Tq]. The following table was created using the M/M/s birth-death model and the R software program by giving the estimated values to these parameters.
As it can be understood from Table-2, during a PGM attack on a target protected by 4 Pantsir-S2 units, if the number of PGMs required to reach the target is between 25 <λ<30, the engagement capacity (density) will be between 0.781<ρ<0.938. If λ=25, then Lq=2.011, meaning the number of PGMs that have not been engaged yet (the number of PGMs waiting in the queue for engagement). If Wq=4.8 sec, it shows the expected time for engagement in the queue. Especially in the case of λ=30 PGMs, it is seen that at least Lq=12.95~13 PGMs will wait in the queue for engagement, and the average waiting time of these PGMs will be approximately Wq=25.95~26 seconds. Naturally, PGMs that have not yet been engaged (waiting in the queue) will not remain in the air, like a market customer, and the target will be destroyed in a few seconds. If λ>32 (in case of using 32 PGMs or more), the system will be completely saturated (ρ>1). Naturally, real war conditions are very different, and the theoretical figures described here may not reflect reality. For example, it may be possible to use decoys instead of real PGMs, or the rapid engagement capability of the Pantsir units can be abbreviated with remote electronic jamming. For example, if Pantsir’s engagement rate per minute is reduced to 2-3 (such as µ=2), the system can be saturated using a much smaller number of PGMs or decoys. Using low RCS munitions such as Delilah, decoys, electronic countermeasures (ECM), and combat tactics such as surprise attacks may play a critical role in Israel’s success against Pantsir systems in Syria in recent years.
Conclusion and Suggestions
In peacetime, the existence and models of air defense and other passive interception systems owned by hostile countries should be identified, and countermeasures should be developed against these systems. In short, it is necessary to have a robust intelligence network and advanced E.W. systems to decrease the effectiveness (reducing the probability of interception) of enemy Air Defense Systems. These E.W. systems include long-range electronic support and countermeasure systems for signal intelligence (SIGINT), land/ship-based electronic support/countermeasure systems, or signal blocking devices (jammers). Returning to the matrix above in Table-3, when we reduce the probability of successful interception of the air defense system from 75% to 50%, the number of PGMs required for at least one hit with a 95% success rate decreases from 10 to 4. Likewise, in addition to the E.W. systems, air-launched decoy missiles such as the ADM-160 MALD (Miniature Air-Launched Decoy/USA) and ITALD (Improved Tactical Air-Launched Decoy/Israel) can also be deployed to trick hostile Air Defense Systems into spending their missiles and ammunition on false targets instead of real targets. Thus, real munitions can be used in the second wave that will follow immediately.
Various factors such as stealth-configured specialized structure geometry and the use of RAM paint and composite materials should also be considered to reduce the radar cross-sections of cruise missiles like SOM as much as possible. Secondly, cruise missiles with advanced guidance systems such as SOM may be upgraded with new features such as swarming capability, networking, and smart flight management systems that can monitor each missile’s status and automatically direct them to another nearby target that is not hit yet.
While countries such as the USA and Israel are developing different sized guided munition options, they have started to prioritize small diameter guided munitions. The USAF and US NAVY are currently investing in 120kg SDB (Small Diameter Bomb) munitions that use two or three different types of guidance, such as GBU-39 (SDB-I) and GBU-53 (SDB-II). Thanks to their control surfaces and guidance systems, these bombs can reach a range of 100 km when launched at medium-high altitudes. An F-16 aircraft can carry a total of 8 SDB-I/II ammunition with two BRU-61 ejector racks, each of which can be loaded with four bombs. Similarly, Israel has developed Spice 250 munition with multiple guidance (INS/GPS and E/O guidance) from the Spice family. It weighs 250lb (122kg) and a total of eight bombs can be carried by an F-16 aircraft.
In parallel with these developments, Turkey is also developing a small-diameter/light-weight bomb with similar guidance characteristics as the US GBU-39 SDB-I munition. Co-developed by Aselsan/SAGE, this munition, which weighs around 125kg, will have a range of 100km when launched from high altitudes thanks to its diamond-shaped folded wings like the GBU-39 SDB-I bomb. Another significant advantage of using SDBs is that it allows carrying a higher amount of munition, enabling multiple target engagements in a single sortie.
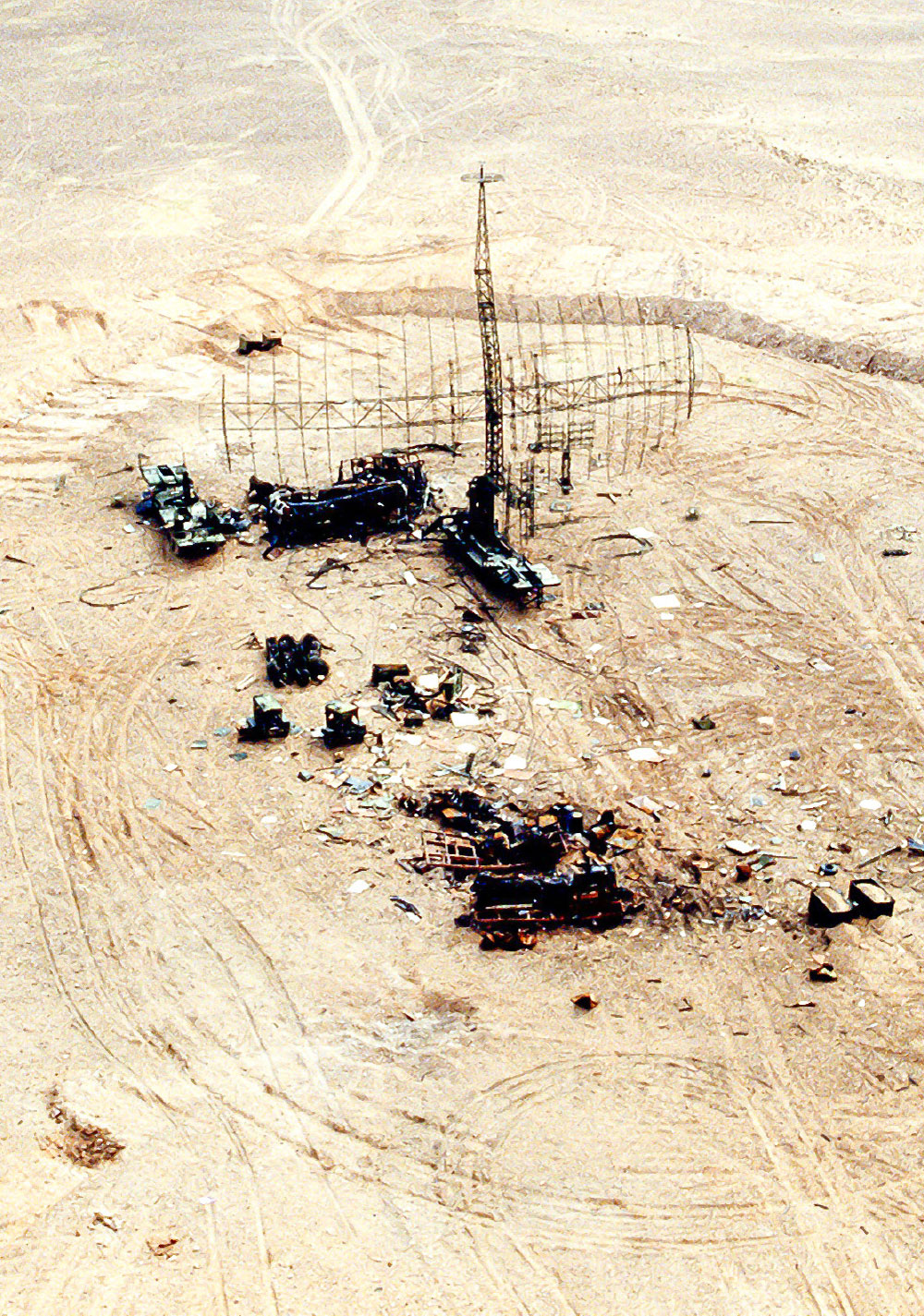
Furthermore, to provide solutions at the operational level, it is necessary to carefully monitor the swarm UAVs and smart munitions that are still in the R&D process. With the introduction of this technology, air defense will no longer be possible with conventional missile/gun systems. It will be inevitable for Air Defense Systems to adapt directed-energy weapons that utilize lasers or electromagnetic (railgun) technologies to counter swarm drone attacks.
On the other hand, in peacetime, PGM stocks should be prepared in different varieties (short, medium, or long-range) and numbers according to the priority and type (such as hard targets, soft targets, or fortifications) of targets to be destroyed. For example, while stocking a large amount of LGB/JDAM type short-range (under 20km) munitions, it is also necessary to have a certain number of medium-range (20-100km) PGMs, and finally, a sufficient number of long-range (over 100km) cruise missiles (air, ground, or sea-launched) are required to hit very high-value critical targets protected by multi-layered Air Defense Systems
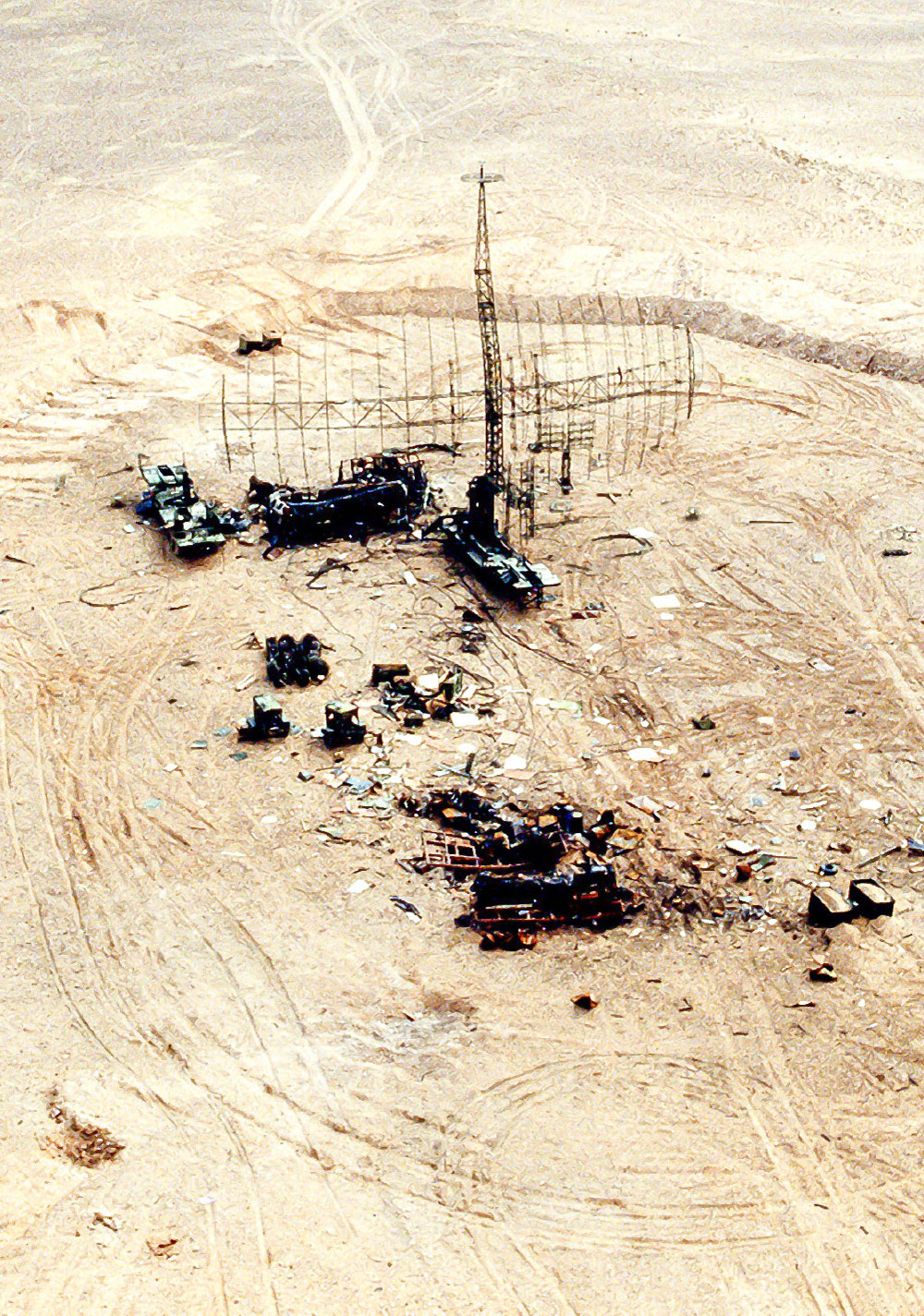
An Analytical Perspective on the Competition Between Air Defense Systems and Guided Air-to-Ground Munitions
Dr. Feridun TAŞDAN - Western Illinois University
